For the first time, scientists have observed a collection of particles, also known as a quasiparticle, that’s massless when moving one direction but has mass in the other direction. The quasiparticle, called a semi-Dirac fermion, was first theorized 16 years ago, but was only recently spotted inside a crystal of semi-metal material called ZrSiS. The observation of the quasiparticle opens the door to future advances in a range of emerging technologies from batteries to sensors, according to the researchers.
The team, led by scientists at Penn State and Columbia University, recently published their discovery in the journal Physical Review X.
“This was totally unexpected,” said Yinming Shao, assistant professor of physics at Penn State and lead author on the paper. “We weren’t even looking for a semi-Dirac fermion when we started working with this material, but we were seeing signatures we didn’t understand—and it turns out we had made the first observation of these wild quasiparticles that sometimes move like they have mass and sometimes move like they have none.”
A particle can have no mass when its energy is entirely derived from its motion, meaning it is essentially pure energy traveling at the speed of light. For example, a photon or particle of light is considered massless because it moves at light speed. According to Albert Einstein’s theory of special relativity, anything traveling at the speed of light cannot have mass.
In solid materials, the collective behavior of many particles, also known as quasiparticles, can have different behavior than the individual particles, which in this case gave rise to particles having mass in only one direction, Shao explained.
Semi-Dirac fermions were first theorized in 2008 and 2009 by several teams of researchers, including scientists from the Université Paris Sud in France and the University of California, Davis. The theorists predicted there could be quasiparticles with mass-shifting properties depending on their direction of movement—that they would appear massless in one direction but have mass when moving in another direction.
Sixteen years later, Shao and his collaborators accidentally observed the hypothetical quasiparticles through a method called magneto-optical spectroscopy. The technique involves shining infrared light on a material while it’s subjected to a strong magnetic field and analyzing the light reflected from the material. Shao and his colleagues wanted to observe the properties of quasiparticles inside silver-colored crystals of ZrSiS.
The team conducted their experiments at the National High Magnetic Field Laboratory in Florida. The lab’s hybrid magnet creates the most powerful sustained magnetic field in the world, roughly 900,000 times stronger than the Earth’s magnetic field. The field is so strong it can levitate small objects such as water droplets.
The researchers cooled down a piece of ZrSiS to -452°F—only a few degrees above absolute zero, the lowest possible temperature—and then exposed it to the lab’s powerful magnetic field while hitting it with infrared light to see what it revealed about the quantum interactions inside the material.
“We were studying optical response, how electrons inside this material respond to light, and then we studied the signals from the light to see if there is anything interesting about the material itself, about its underlying physics,” Shao said. “In this case, we saw many features we’d expect in a semi-metal crystal and then all of these other things happening that were absolutely puzzling.”
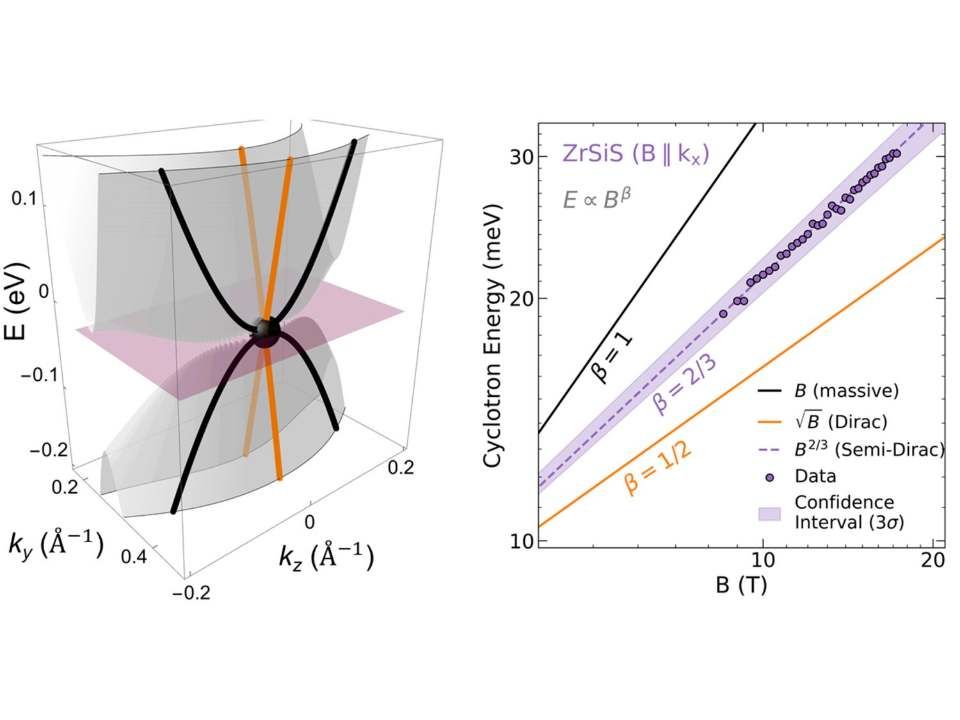
When a magnetic field is applied to any material, the energy levels of electrons inside that material become quantized into discrete levels called Landau levels, Shao explained. The levels can only have fixed values, like climbing a set of stairs with no little steps in between. The spacing between these levels depends on the mass of the electrons and the strength of the magnetic field, so as the magnetic field increases, the energy levels of the electrons should increase by set amounts based entirely on their mass—but in this case, they didn’t.
Using the high-powered magnet in Florida, the researchers observed that the energy of the Landau level transitions in the ZrSiS crystal followed a completely different pattern of dependence on the magnetic field strength. Years ago, theorists had labeled this pattern the “B2/3 power law,” the key signature of semi-Dirac fermions.
To understand the bizarre behavior they observed, the experimental physicists partnered with theoretical physicists to develop a model that described the electronic structure of ZrSiS. They specifically focused on the pathways on which electrons might move and intersect to investigate how the electrons inside the material were losing their mass when moving in one direction but not another.
“Imagine the particle is a tiny train confined to a network of tracks, which are the material’s underlying electronic structure,” Shao said. “Now, at certain points the tracks intersect, so our particle train is moving along its fast track, at light speed, but then it hits an intersection and needs to switch to a perpendicular track. Suddenly, it experiences resistance, it has mass. The particles are either all energy or have mass depending on the direction of their movement along the material’s ‘tracks.'”
The team’s analysis showed the presence of semi-Dirac fermions at the crossing points. Specifically, they appeared massless when moving in a linear path but switched to having mass when moving in a perpendicular direction. Shao explained that ZrSiS is a layered material, much like graphite that is made up of layers of carbon atoms that can be exfoliated down into sheets of graphene that are one atom thick. Graphene is a critical component in emerging technologies, including batteries, supercapacitors, solar cells, sensors and biomedical devices.
“It is a layered material, which means once we can figure out how to have a single layer cut of this compound, we can harness the power of semi-Dirac fermions, control its properties with the same precision as graphene,” Shao said. “But the most thrilling part of this experiment is that the data cannot be fully explained yet. There are many unsolved mysteries in what we observed, so that is what we are working to understand.”
Reference: Yinming Shao et al, Semi-Dirac Fermions in a Topological Metal, Physical Review X (2024). DOI: 10.1103/PhysRevX.14.041057
[…] Read more […]