Astronomers tallying up all the normal matter—stars, galaxies and gas—in the universe today have come up embarrassingly short of the total matter produced in the Big Bang 13.6 billion years ago. In fact, more than half of normal matter—half of the 15% of the universe’s matter that is not dark matter—cannot be accounted for in the glowing stars and gas we see.
New measurements, however, seem to have found this missing matter in the form of very diffuse and invisible ionized hydrogen gas, which forms a halo around galaxies and is more puffed out and extensive than astronomers thought.
The findings not only relieve a conflict between astronomical observations and the best, proven model of the evolution of the universe since the Big Bang, they also suggest that the massive black holes at the centers of galaxies are more active than previously thought, fountaining gas much farther from the galactic center than expected—about five times farther, the team found.
“We think that, once we go farther away from the galaxy, we recover all of the missing gas,” said Boryana Hadzhiyska, a Miller postdoctoral fellow at the University of California, Berkeley, and first author of a paper reporting the findings. “To be more accurate, we have to do a careful analysis with simulations, which we haven’t done. We want to do a careful job.”
“The measurements are certainly consistent with finding all of the gas,” said her colleague, Simone Ferraro, a senior scientist at Lawrence Berkeley National Laboratory (Berkeley Lab) and at UC Berkeley who saw hints of this extensive ionized hydrogen halo in analyses published three years ago.
The results of the study, co-authored by 75 scientists from institutions around the world, have been presented at recent scientific meetings, posted as a preprint on arXiv and are undergoing peer review at the journal Physical Review Letters. Hadzhiyska and Ferraro are researchers at the Berkeley Center for Cosmological Physics in UC Berkeley’s Department of Physics, as well as at Berkeley Lab.
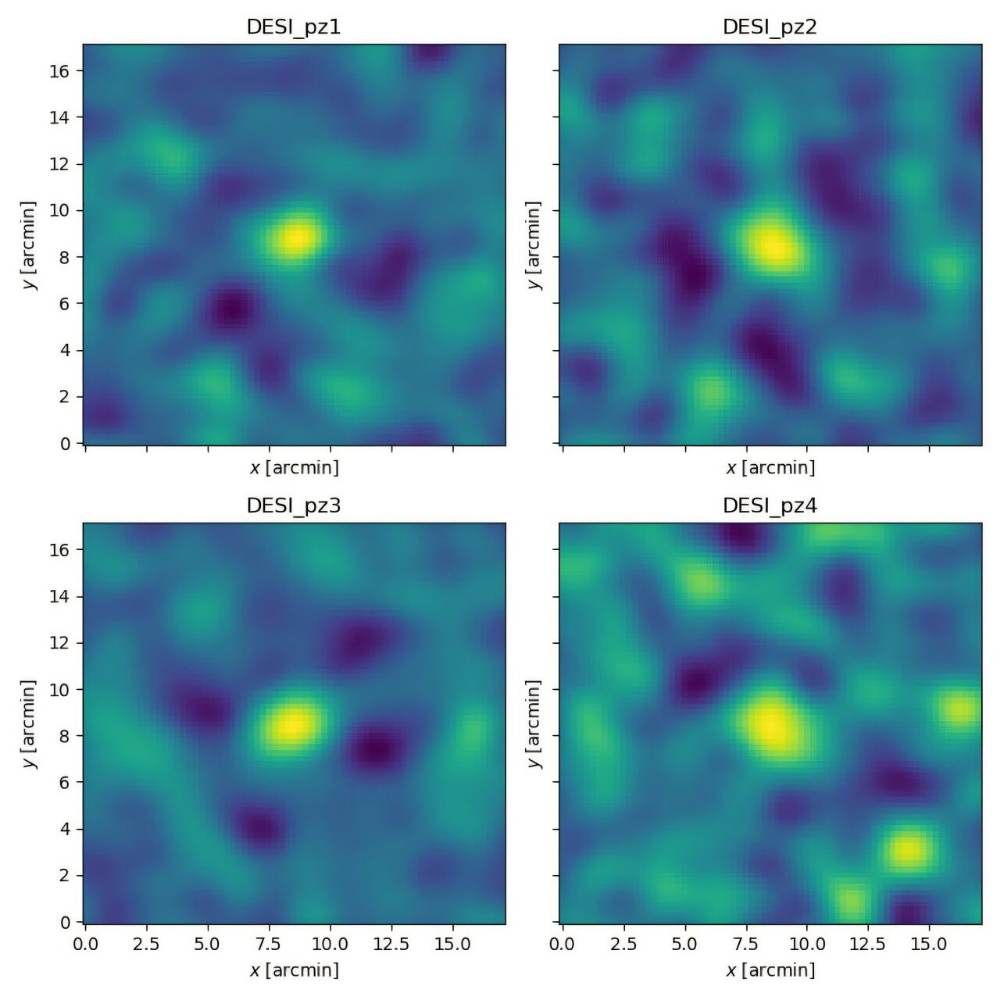
While the still mysterious dark matter makes up the bulk—about 84%—of matter in the universe, the remainder is normal matter. Only about 7% of normal matter is in the form of stars, while the rest is in the form of invisible hydrogen gas—most of it ionized—in galaxies and the filaments that connect galaxies in a kind of cosmic network.
The ionized gas and associated electrons strung out in this filament network are referred to as the warm-hot intergalactic medium, which is too cold and too diffuse to be seen with the usual techniques at astronomers’ disposal, and therefore has remained elusive until now.
In the new paper, the researchers estimated the distribution of ionized hydrogen around galaxies by stacking images of approximately 7 million galaxies—all within about 8 billion light-years of Earth—and measuring the slight dimming or brightening of the cosmic microwave background caused by a scattering of the radiation by electrons in the ionized gas, the so-called kinematic Sunyaev-Zel’dovich effect.
“The cosmic microwave background is in the back of everything we see in the universe. It’s the edge of the observable universe,” Ferraro said. “So you can use that as a backlight to see where the gas is.”
The galaxy images used—all luminous red galaxies—were collected by the Dark Energy Spectroscopic Instrument (DESI) on the Mayall 4-meter Telescope at Kitt Peak National Observatory in Tucson, Arizona. The instrument, built by a collaboration headquartered at Berkeley Lab, is surveying tens of millions of galaxies and quasars to construct a 3D map spanning the universe out to 11 billion light years from Earth in order to measure the effect of dark energy on the expansion of the universe.
Measurements of the cosmic microwave background (CMB) around these galaxies were made by the Atacama Cosmology Telescope (ACT) in Chile, which made the most accurate measurements to date of the CMB before it was decommissioned in 2022.
The analysis was done in collaboration with Bernardita Ried Guachalla, a graduate student at Stanford University; Emmanuel Schaan, a staff scientist at the SLAC National Accelerator Laboratory in Menlo Park; and the DESI and ACT teams.
Astronomers have generally thought that massive black holes at the centers of galaxies expel gas in jets of material only during their formative years, when the central black hole is gobbling up gas and stars and producing lots of radiation. This makes them stand out as what astronomers call active galactic nuclei (AGN), or quasars.
If, as the new study suggests, the ionized hydrogen halo around galaxies is more diffuse, but also more extensive, than thought, this implies that the central black holes may actually become active at other times in their lives.
“One problem we don’t understand is about AGNs, and one of the hypotheses is that they turn on and off occasionally in what is called a duty cycle,” said Hadzhiyska.
Astronomers refer to the expulsion of gas and its subsequent fall back into the galactic disk as feedback that regulates the formation of new stars throughout the galaxy. Ferraro, Schaan and their colleagues reported hints of more extended feedback in previous work in 2020, when Schaan was a postdoctoral fellow at Berkeley Lab.
But the new work incorporates more galaxies and produces a more precise measurement. Subsequent work by Ried Guachalla confirmed the findings with the DESI spectroscopic sample, and was able to study the gas in more nearby galaxies, highlighting that the gas is not distributed uniformly around them, but follows “cosmic filaments” that permeate the universe.
Hadzhiyska noted that current simulations of galaxy evolution will need to incorporate this more vigorous feedback in their models. Some new models are already doing so to produce stronger simulations in better agreement with the new data.
The identification of the missing matter, or baryons, in the universe also has implications for other aspects of cosmic evolution.
“Knowing where the gas is has become one of the most serious limiting factors in trying to get cosmology out of current and future surveys. We’ve kind of hit this wall, and this is the right time to address these questions,” Ferraro said. “Once you know where the gas is, you can ask, ‘What’s the consequence for cosmological problems?'”
For one, the expulsion of gas from the cores of these massive galaxies challenges the assumption that gas follows dark matter, Hadzhiyska said. Underestimating this gas expulsion can introduce inconsistencies into cosmological models, while the new results may actually resolve some issues about how clumpy the universe is.
“There are a huge number of people interested in using our measurements to do a very thorough analysis that includes this gas,” she said. “People in astronomy care a lot about it for understanding galaxy formation and evolution.”
The technique the team used, the kinematic Sunyaev-Zel’dovich effect, could also be used to probe the early universe, Hadzhiyska said. This could provide insight into the large-scale structure of the universe and the laws of physics in the early universe and allow scientists to test gravity and general relativity.
Reference: B. Hadzhiyska et al, Evidence for large baryonic feedback at low and intermediate redshifts from kinematic Sunyaev-Zel’dovich observations with ACT and DESI photometric galaxies, arXiv (2024). DOI: 10.48550/arxiv.2407.07152